Introduction of the Researches
Mathematical Biology Laboratory (Usami Laboratory)
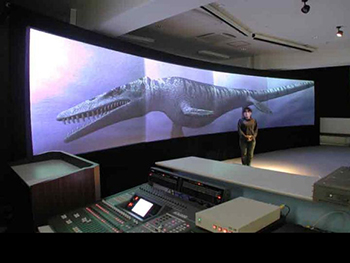
Autonomous driving brings not only safety but also economic benefit for industries. Japanese car companies provide developed sensors for cars, but integrations of those elementary equipments have achieved by American companies. Our laboratory imported an autonomous driving system of Comma.ai for Japanese automobile environment. We found out that US system can be directly applied to Japanese roads. We have achieved round trip from Tokyo to Osaka on April, 2018. Slight difference between US-left handle system and Japanese one have been found. We try to fix this problem and the others by changing source chords of the software.
High-energy Particle Observation Labolatory (Udo Laboratory)
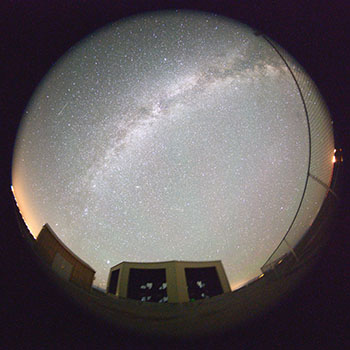
About 100 years ago, high energy particles which coming from space were discovered and named Cosmic Ray. “Ultra-High-Energy cosmic rays” are especially high energy particles among cosmic rays, those are expected to identify the origin of cosmic ray because the UHE cosmic rays are less susceptible from galactic and extra galactic magnetic field. However, cosmic ray particle which arrive to the earth collide with atmosphere, we can't observe it directly at ground. So we measure primary energy and arrival direction of cosmic ray using phenomena called extended air shower which caused by nuclear interaction between cosmic ray and atmosphere.
We are observing the Ultra-High-Energy cosmic rays in desert of Utah, USA with two types of detectors of Surface particle Detector array (SD array) and Fluorescence Detector telescope (FD telescope). While exploring the origin of cosmic ray with these two kinds of detectors, we are studying development of new particle detector, automation of experimental devices by mechatoronics and calibration of telescope optics etc.
Nano Science Laboratory (Kyakuno Laboratory)
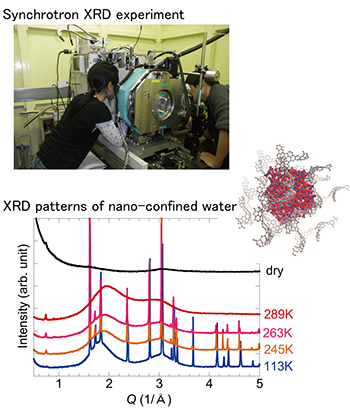
Nano substances often exhibit unusual properties that do not appear in the bulk. They are of significant interest with respect to the basic science, as well as the development of new nanoscale devices. We study on substances in confining geometries of nano structural materials using a variety of experiments and simulations, such as synchrotron X-ray diffraction (XRD) experiments, thermal analyses, electrical measurements, optical measurements, scanning probe microscope observations, and molecular dynamics simulations. The specific examples of our research topics are as follows:
(1) We study on confined fluids, such as water, rare gases, and hydrocarbons, inside nano pores. For example, it was shown that hydrogen-bonded structures of the confined water can be controlled by the size, shape, topology, and hydrophobicity of nano pores. We investigate novel properties and functions of the confined water. The study contributes to the development of high-performance filtration/separation devices and to better understanding of the function of biological channels.
(2) Single wall carbon nanotubes (SWCNTs) have high potential as flexible and lightweight thermoelectric materials. In previous studies, it was found that thermoelectric properties of SWCNT bundles substantially depend on the carrier density and the mixing ratio of semiconducting and metallic SWCNTs. We examine thermoelectric properties of bulk SWCNT materials employing both experiments and simulations. Our goal is to find out the conditions under which bulk SWCNT materials have sufficiently high thermoelectric properties to meet practical use.
Computational Statistical Physics Laboratory (Sasaki Laboratory)
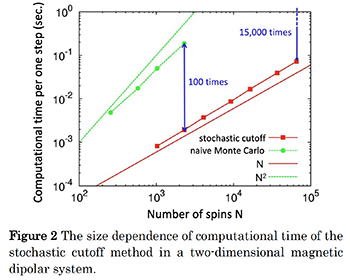
We investigate disordered systems, such as spin glasses and glasses, with statistical mechanics and computer simulations. Spin glass is a random magnet in which ferromagnetic and antiferromagnetic interactions coexist randomly. Glass is a metastable state in which molecular arrangement is frozen randomly. Disordered systems often exhibit curious phenomena which are not observed in regular systems. Temperature chaos, which is a phenomenon that the equilibrium state chaotically changes with temperature, is one of them (see Fig. 1). One of the purposes of our study is to clarify the mechanism of these curious phenomena in disordered systems.
We have also developed efficient Monte-Carlo methods for disordered systems and long-range interacting systems. A stochastic cutoff method is one of them. This method enables us to reduce computational time for long-range interacting systems without any approximations (see Fig. 2). As an application research of the stochastic cutoff method, we have also studied thermal effects in nanomagnets.
Space Environment Laboratory (Shimizu Laboratory)
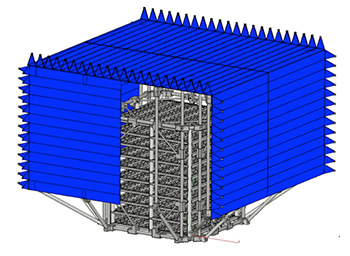
To investigate elementary particle phenomena in space environment, we develop observation instruments equipped on satellites and high-altitude balloons. Dark matter, unidentified mass component in the Universe, is thought to be non-baryonic particles and rarely interact with ordinary matter. Since dark matter particles are predicted to annihilate or decay into antiparticles such as antideuterons and antiheliums, observation of cosmic-ray antiparticles is one of the most promising way to search dark matter.
Currently we develop an antiparticle spectrometer to be mounted on Antarctica long duration balloons. We also observe high energy cosmic rays with a electromagnetic calorimeter on the International Space Station.
Cosmic-ray Radiation Measurement Laboratory (Tamura Laboratory)
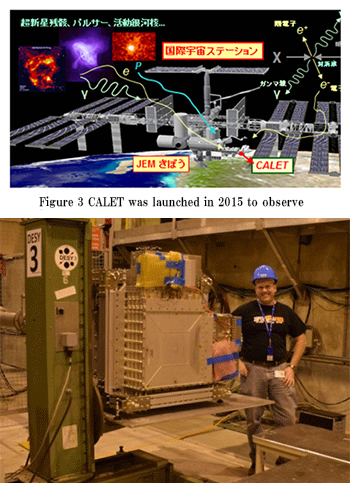
High energy phenomena like supernova explosions and back holes occur in the universe. In such environments, electrons and protons must be accelerated up to very high energy closing to the light speed. They are called “cosmic rays” and can be observed at the Earth. I have been developing detectors to measure radiations and particles to observe them at altitudes of balloons or the International Space Station.
In balloon experiments, payloads of about 300 kg are brought up to altitudes around 40 km where the atmospheric pressure is reduced to a few hPa. Cosmic rays can be observed in such low pressure environment before they are attenuated by the atmosphere. In order to observe cosmic-ray electrons, our collaboration group carried out several balloon observations in Japan and also performed a long duration balloon observation for 2 weeks in Antarctica.
Successful results obtained from these balloon experiments have led to a project to observe cosmic rays at the International Space Station. I am involved in the JAXA project to develop the CALET instrument. In development from the very beginning, detector structure designs, developments of front end electronics and data taking system, simulations to optimize detector capabilities, tests to evaluate performance of instruments, and so on are needed. Originality to derive scientific results from taken data is also very important for data analyses.
Our measurements of radiations and particles have a lot of techniques which can be applied to measurements of environmental radiations and medical instruments such as PET (Positron Emission Tomography).
Theoretical Quantum Physics Laboratory (Nishino Laboratory)
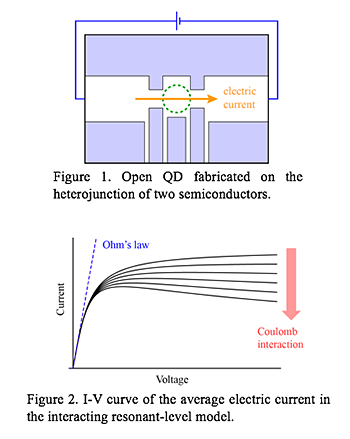
Nanoscience has attracted much interest in various fields of physics, chemistry, and engineering. In particular, quantum transport in nanoscale devices is a rapidly developing field of condensed matter physics. For devices smaller than the coherent length, quantum effects appear in the electron transport, which cannot be described by classical Ohm’s law. To analyze quantum transport theoretically, we must treat nonequilibrium steady states in open quantum systems. The Landauer formula can be used to calculate electrical conductance with the transmission eigenvalues of the scattering matrix, which implies that the nonequilibrium steady states are scattering eigenstates. However, the formula has been restricted to non-interacting cases.
We study the quantum transport of interacting electrons in open quantum systems. Let us consider the nanoscale device shown in Figure 1. The blue area in Figure 1 is negatively charged, and hence electrons are localized in the small area indicated by the dashed green circle, which is called a quantum dot (QD). We must consider the Coulomb repulsion for the electrons localized in the QD. Recently, we have proposed an extension of the Landauer formula for these QD systems that include interactions. Through constructing exact many-electron scattering eigenstates, we have obtained an analytical form of the average electric current for the interacting resonant-level model under bias voltages. Figure 2 shows the I-V curve of the average electric current. The electric current is suppressed for large bias voltages, which is the negative differential conductance originating from the Coulomb interaction.
Astroparticle Laboratory (Hibino Laboratory)
Astroparticle physicists strive to find the answers to some of the most exciting questions about the Universe. What is the origin of cosmic rays? Where does the acceleration of ultra-high- energy cosmic rays originate? What is the role of violent astrophysical processes? Can we detect dark matter?
The laboratory’s main study is gamma-ray astrophysics, extending from mega-electronvolts (MeV) to peta-electronvolts (PeV), and covers a wide range of phenomena in particle physics and astrophysics, providing one of the most active fields of modern astroparticle physics. Moreover, the laboratory tackles challenges in technology and computing. The Tibet Experiment which is a collaboration between China and Japan, aims to observe cosmic rays and gamma rays with energies greater than 1013 eV at Yangbajing (4.300m above sea level) in Tibet, China.
Electronic Materials Physics Laboratory (Matsuda Laboratory)
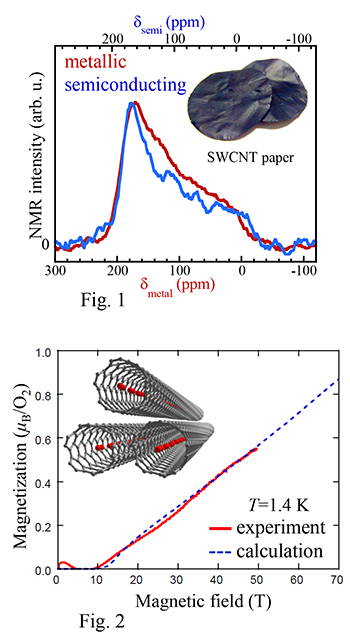
1) Carbon nanomaterials are promising for the development of new technologies owing to their unique electronic, thermal, and elastic properties. SWCNTs can behave as metals or semiconductors depending on their helicity and diameter. By using a nuclear magnetic resonance (NMR) technique, we study the electronic states of SWCNTs and other carbon nanomaterials. (Fig. 1. 13C-NMR spectra obtained for metallic and semiconducting SWCNTs. The inset shows a highly purified SWCNT paper.)
(2) SWCNTs serve as model systems for confined molecules in cylindrical cages a few molecules wide. We are exploring the properties of molecular materials confined to the nanoscale cages of network solids. (Fig. 2. Magnetization curves for oxygen adsorbed inside SWCNTs. The inset shows a schematic of the alignment of oxygen molecules encapsulated in the SWCNTs.)
(3) One of the constituent materials of cement, insulating 12CaO・7Al2O3, with a subnanoscale structure becomes metallic by the heat treatment. We study the metallization mechanism of 12CaO・7Al2O3. (Fig. 3. Structure of an empty cage of 12CaO・7Al2O3 and the temperature dependence of the 27Al nuclear spin-lattice relaxation rate, T1-1.)